Bacterial organelle pores work independent of shape
Article Highlights
- Researchers from the Plant Research Laboratory at Michigan State University discovered that bacterial microcompartments will function the same, regardless of their shape
- The findings were published in ACS Omega
- Computational experiments on bacterial microcompartments can greatly reduce their computing power by simulating smaller, flat chunks of the BMC instead of the whole structure.
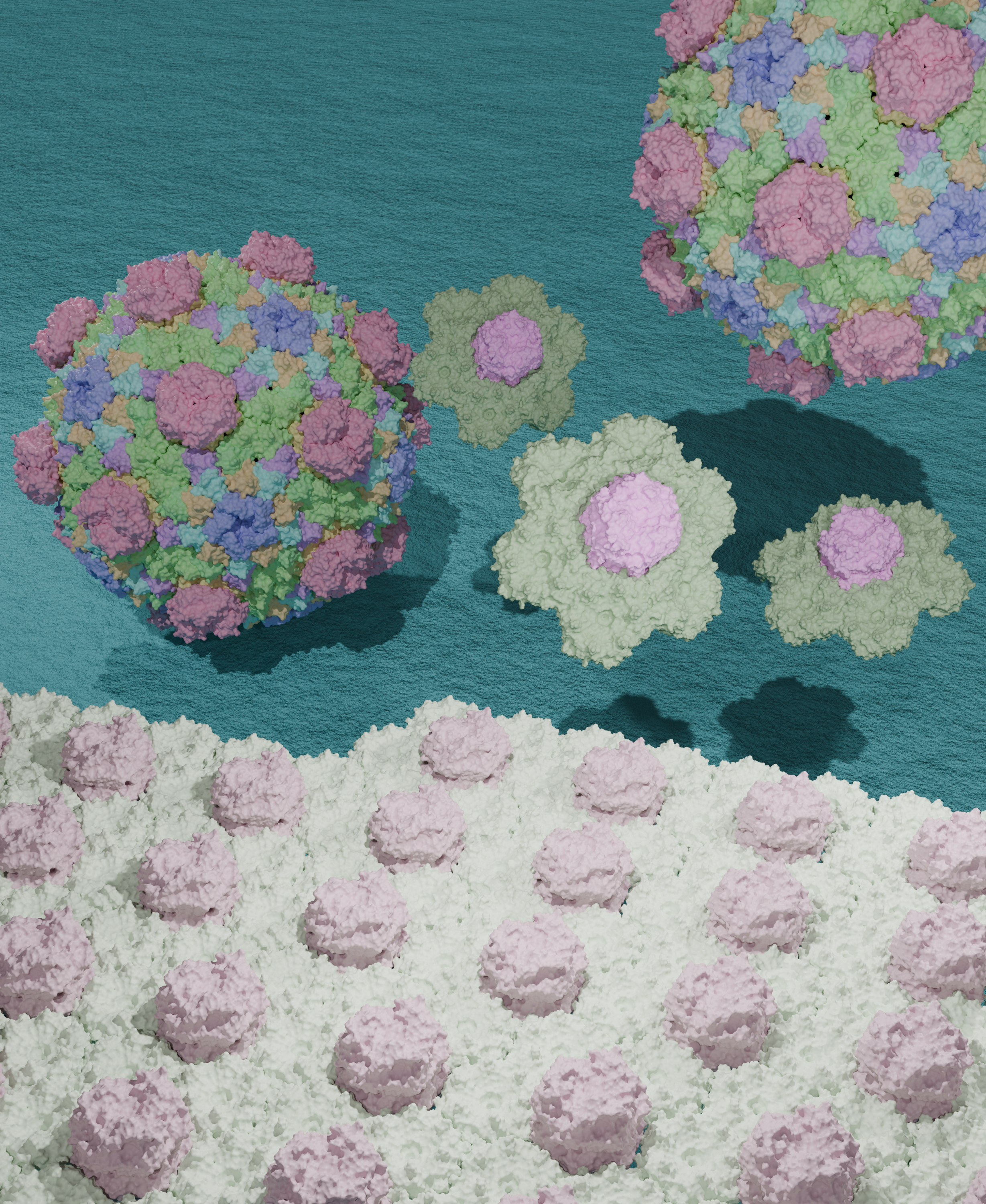
By Saad Raza
Within a bacterium’s single cell, hundreds of reactions and syntheses are underway. Some can be volatile, yet they are crucial to the bacteria’s function and need to be kept safely isolated from other parts of the cell.
Some of those reactions take place in a structure called a bacterial microcompartment, or BMC: a pore-covered organelle that looks similar to the polyhedral dice used in board games. But in the lab, BMCs can take on a flattened sheet shape.
Researchers have now confirmed that the BMC pores work the same regardless of what configuration a BMC is in — and understanding how those pores work is vital to employing BMCs for human use, such as drug synthesis. The team published a paper about their work in the journal ACS Omega.
“We are trying to use this architecture to do new, cool chemistry that we would like it to do, not what the bacteria wants,” said Josh Vermaas, an assistant professor and researcher in the Michigan State University-Department of Energy Plant Research Laboratory, or PRL, and the Department of Biochemistry & Molecular Biology, or BMB.
The features of BMCs make them an enticing structure for scientists who are doing their own molecular synthesis, particularly ones that involve harmful intermediates. Looking far into the future, Vermaas said, BMCs could be used to make cancer drugs, or process toxic waste.
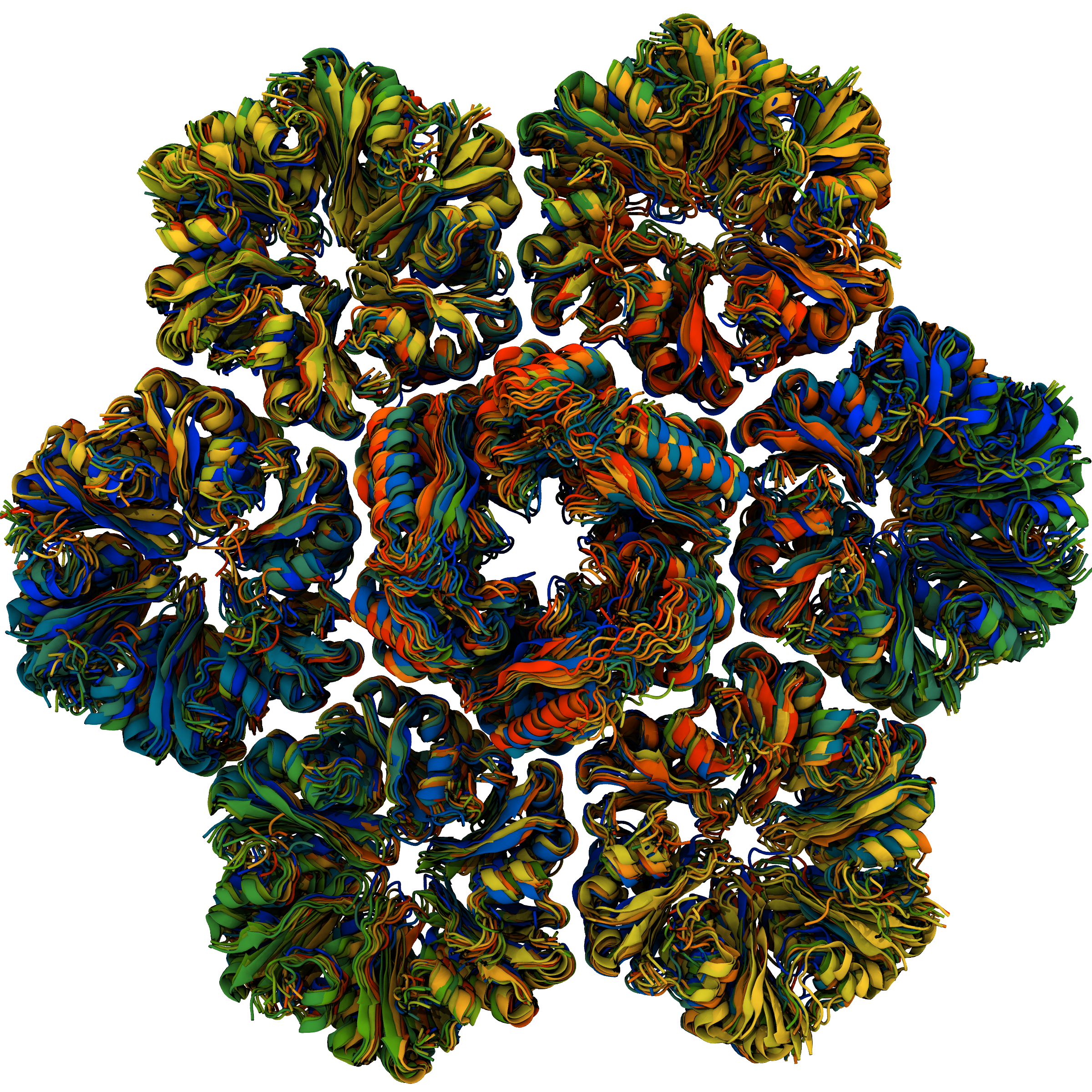
By Josh Vermaas
BMCs act like a high-security laboratory for volatile reactions, wherein molecules are converted from one form to another, while keeping them contained and separate from the rest of the bacterium. And, because the reactions within a BMC are all in one spot, they become concentrated. That means these reactions can happen faster.
Because of these unique features, researchers have been studying BMCs for several years in pursuit of molecular synthesis. Some research is done in a wet lab, where BMCs are observed directly. Other research is done computationally, using computer programs to simulate how the organelles behave or how molecules interact with them. But simulating an entire BMC shell takes an incredible amount of computational power and energy.
Vermaas and postdoctoral researcher Saad Raza wanted to know if, instead, they could simulate a small chunk of a BMC and still get the same results. To do that, they compared how pore properties would change in a curved orientation as compared to a flattened one.
The shell of a BMC is made up of tiles called hexamers and trimers, the latter of which hadn’t been used in a simulation like this, Vermaas said. That’s exciting because trimers have a larger pore size, meaning larger molecules can fit through, Raza added.
At the center of each tile is a pore. Raza used a computer program that simulated the atoms of a BMC’s protein-based tiles. But instead of testing the entire BMC shell through simulations, Raza tested a curved chunk of tiles — a proxy for the curved shell — and a chunk of flattened tiles to represent a sheet. Then, he applied Newton’s laws of physics to those atoms, allowing the team to see how the atoms behave in different circumstances. They focused on the pore specifically, hoping to tease apart any differences in diameter between the two shapes of BMC chunks.
By Kara Headley
Cheryl Kerfeld, whose group uses wet lab approaches to the study of BMCs, said Raza and colleagues’ findings will help them to make designer BMCs with the necessary pore permeability much faster.
“Saad’s analyses will help us hone in on the structures we need to build much faster,” said Kerfeld, Hannah Distinguished Professor in the PRL and BMB, and author on the paper. Kerfeld is also the director of the Center for Catalysis in Biomimetic Confinement, which funded the majority of this work. “It’s a great example of teamwork between theorists and experimentalists.”
By successfully flattening the BMC tile assemblies, the team also showed that computational experiments involving the pores will still be accurate even at a smaller size. That smaller size saves hundreds of thousands of atoms from being simulated, saving time and energy toward computational power.
“The big picture is that the pore dynamics are independent,” Raza said. “This makes it easier to reduce our models, and it helps in understanding it computationally and experimentally.”
This research was funded by the Center for Catalysis in Biomimetic Confinement, an Energy Frontier Research Center funded by the U.S. Department of Energy, Office of Science, Basic Energy Sciences, under Award DE-SC0023395. Additional funding includes the Office of Science of the U.S. Department of Energy; the National Institutes of Health; the U.S. Department of Energy, Office of Basic Energy Sciences under Grant Number DE-FG02-91ER20021. This research used resources of the National Energy Research Scientific Computing Center (NERSC) and the Institute for Cyber-Enabled Research at Michigan State University.