Taming electrons with bacteria parts and a little 'blood' - a new synthetic biology system
Video summary in 90 seconds
Electrons are tough to pin down in biology. Trying to guide one to go where you want it is as unpredictable as tipping a bean machine over and guessing where individual beans will end up.
Learning how to harness electrons is no fool’s errand, though. Because, when electrons move, they are the electricity that powers life.
Electrons power the production of fuel and medicine. Electron movement is behind expand iconphotosynthesis, our main source of food, and combustion. Moving electrons are the definition of an electric current, which is why you can read this story.
In a new study, scientists at the MSU-DOE Plant Research Laboratory report a new synthetic system that could guide electron transfer over long distances. The new system is made up of two components plucked from nature. One is a expand iconprotein from bacteria and the other a molecule found in our blood.
Iron-containing molecules that make your blood red
Nature has figured out how to tame electrons. The trick is to split up their journeys into short pit stops that are easier to manage. Electrons then hop between stops as they are guided towards some final destination.
One of these natural pit stops is the heme, a molecule that contains iron. It is what gives our blood its color, and it is found in many other biological molecules.
"In nature, multiple hemes have to be closely positioned and angled precisely to allow for fast electron hops. The hemes are fixed in place by attaching to protein structures," says Jingcheng Huang, a former graduate student in the labs of Danny Ducat and David Kramer. "Otherwise, if the distances between hemes become to large, an electron will hop out of control. It is lost."
Artificial pit stops
Since hemes are found in almost all living beings, they can associate with many types of proteins. So the science team used the protein BMC-H, from expand iconbacteria, to build their artificial electron pit stops.
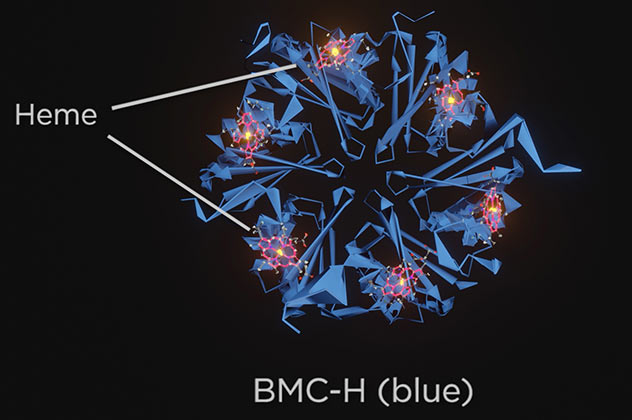
By MSU-DOE Plant Research Laboratory, 2020
The team identified four possible locations the heme can dock into. Specifically, the alpha helical region was the most promising host area.
"We didn't have to modify the BMC-H protein much," Jingcheng says. "With only three amino acid substitutions, we can get a heme binding tightly to it. Because the modification is minimal, the protein's shape and functions remain intact."
Why BMC-H?
“It is known to assemble with other proteins of its type into large and defined structures. Groups of BMC-H have been seen to cluster into flat sheets, tunnels, or swiss roll shapes. So if we find an easy way to attach hemes to these large structures, we could facilitate electron transfer over long distances," Jingcheng says.
The scientists have managed to produce these larger structures with hemes attached to them. Moreover, they can produce them 'naturally' inside of bacteria cells, which saves resources.
"We’d like to optimize this system into a functional 'nanowire’,” Jingcheng, adds. “Someday, it could funnel electrons to power the production of new medicines, or biofuels or electronic devices made of biogoo. The possibilities are endless."
"The exciting part is that we played with what nature has already figured out. We took a protein that self-assembles into large structures but doesn't bind hemes and functionalized it so that it hosts them,” Jingcheng muses. “Otherwise, if we had created a system from scratch, we would have add extra layers of difficulty. That's the essence of synthetic biology, taking natural ingredients and re-configuring them in new, unseen ways."
The study is published in Frontiers in Bioengineering and Biotechnology.
This work was funded by a National Science Foundation Emerging Frontiers grant and the US Department of Energy, Office of Basic Energy Sciences. Collaborators included members of the Kerfeld and Kramer labs at the MSU-DOE Plant Research Laboratory.
By Igor Houwat, Jingcheng Huang