Project A: Photosynthesis in real environments
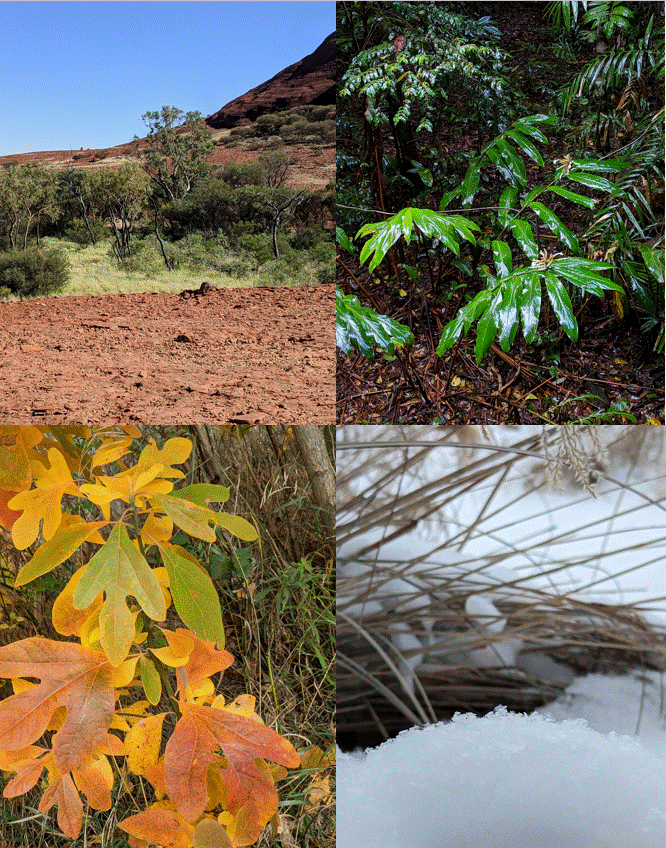
Photosynthetic organisms have evolved to overcome the challenges imposed by constantly
changing and extremely diverse environmental conditions. We aim to understand the
biophysical mechanisms that allow photosynthesis to work in these dynamic, real-world
conditions.
Photos: Atsuko Kanazawa
Photosynthesis provides nearly all the energy in our ecosystem, and all of our agriculture. It is also the major mechanism that uptakes CO2 from our atmosphere. Increasing the efficiency and resilience of photosynthesis is essential to improving crop productivity to feed the vast populations and close the global carbon gap needed to prevent runaway climate changes. To do this, we must understand how the photosynthetic reactions and machinery are operating in real, dynamic environments.
Phototrophs – organisms including plants and algae – use sunlight through photosynthesis to power their biochemical needs for cell maintenance, growth and defense. Decades of research in the laboratory have given us many details of photosynthetic machinery and functions, but our work shows that these organisms behave differently under outside the lab setting and in the dynamic environmental conditions of the real world.
Photosynthetic organisms in natural environments are exposed to extremely diverse environmental conditions, including fluctuations in light intensity and quality, temperature and humidity, CO2 availability, nutrient levels and other factors. All of this variability impacts tradeoffs in photosynthetic efficiency or photodamage. Moreover, rapid and unpredictable fluctuations in conditions can be more damaging than more gradual changes. Diverse organisms have evolved multiple mechanisms to overcome these challenges, and these become important under different conditions.
1. Understanding and manipulating ferredoxin-dependent electron distribution within the chloroplast (Benning, Kramer, Schmollinger, Strenkert, Vermaas, Walker)
How the diverse electron carriers in chloroplast channel electron transfer reactions to energy storage in the right products, and away from reactive oxygen species (ROS) production.
2. O2 tolerance of photosynthetic energy storage: Novel roles for redox signaling, the CCM, alternative electron flow and regulation of the thylakoid proton motive force (pmf) (Benning, Kramer, Neofotis, Schmollinger, Strenkert, Walker)
How the phototrophs respond to highly oxidative conditions, such hyperoxia, and what these processes reveal about the balancing of energy capture and photoprotection, and new roles for the algal carbon concentrating mechanism (CCM).
3. An oxidative peroxiredoxin lipid hub for sensing thylakoid redox stress (Benning, Hoh, Froehlich, Kanazawa, Kramer, Nicodemus, Schmollinger, Strand, Strenkert, Walker)
How are oxidative stress and redox status sensed within the thylakoid membrane and lumen, and how do these adjust photosynthetic responses?
4. The mechanisms that govern photosynthesis in the real world (Benning, Ducat, Hu, Kanazawa, Kerfeld, Kramer, Kuhlgert, Lechno-Yossef, Walker)
Under what organisms and under what conditions do the “canonical” (standard) models of photosynthesis break down, and what new mechanisms take over? What are their costs and benefits, and what biological problems did they evolve to solve? How do these mechanisms extend to very diverse organisms, especially cyanobacteria, which have very different antenna systems?
Our goal is to harness new technologies to find the “new biology” that underlies these responses in dynamic environments. Our work cuts across many species of phototrophs (cyanobacteria, green algae and vascular plants) and disciplines, drawing on expertise in photosynthesis, bioinformatics, lipid biochemistry and cellular and structural biology with projects addressing the following specific questions:
This research is one of three core projects funded by the US Department of Energy, Office of Basic Energy Sciences.