Project C: The chloroplast in the cellular context
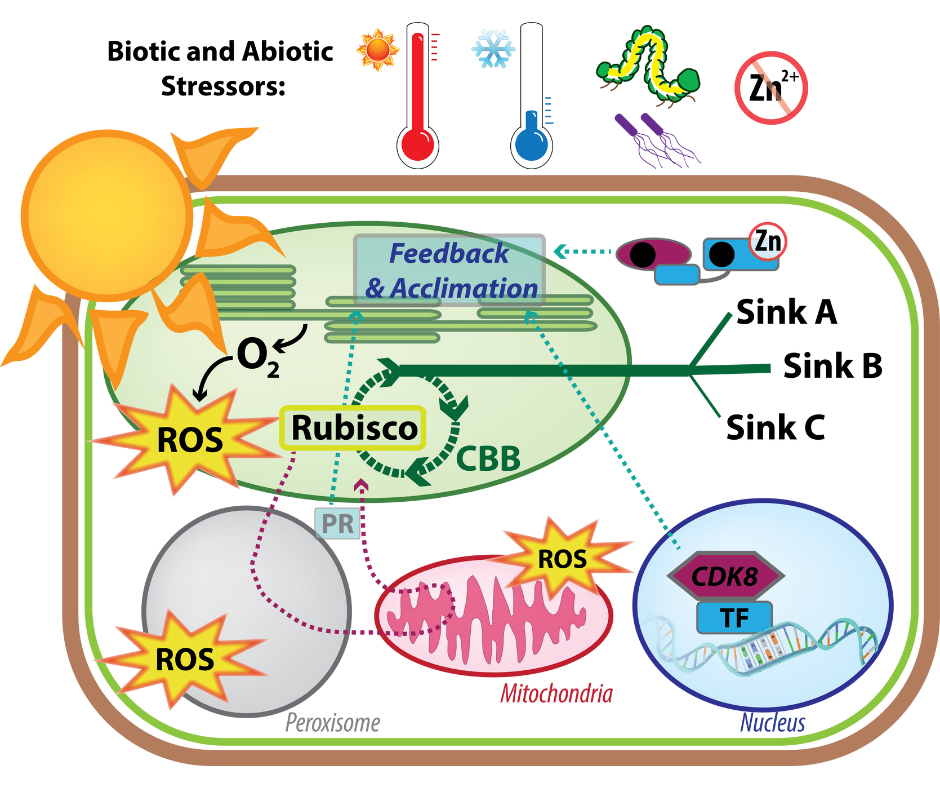
Image by Danny Ducat
Photosynthesis requires the coordination of many different cellular processes simultaneously; inefficiencies in any one process will limit the overall capacity of the plant to convert sunlight energy into fixed carbon and other forms of cellular energy. The textbook example of photosynthesis focuses heavily upon the processes that occur in the chloroplast. Yet, although the core reactions of photosynthesis (e.g., light capture, carbon dioxide fixation) are indeed housed in the chloroplast, there are many other processes that occur outside of the chloroplast that are critical. The projects in this portion of PRL’s work focus on a variety of ways in which the chloroplast communicates with the rest of the cell in order to integrate processes that occur within the chloroplast to the other activities going on in the rest of the cell or even the total plant.
Key questions in the research areas include:
- How does a plant sense an imbalance between chloroplast activities and other processes involved in photosynthesis inside the cell?
- What are the key cellular factors (e.g., proteins) involved in communicating such imbalances?
- Once an imbalance is detected, how does the plant cell coordinate across its different compartments to bring the system back into balance and restore efficiency of photosynthesis?
- Can we leverage knowledge of these cellular pathways to engineer plants with more efficient capture of solar energy?
Projects in this area of PRL research include:
1. The role of photorespiration in balancing photosynthesis across different environments (Brandizzi, Hu, Kramer, Sharkey, Walker)
Photorespiration is usually described as an energetically “wasteful” pathway that happens when the enzyme that fixes carbon dioxide (Rubisco) makes a “mistake.” When Rubisco captures oxygen rather than carbon dioxide, it creates a toxic metabolite that must be processed through a series of energetically expensive reactions that span across multiple cellular compartments, collectively referred to as photorespiration. Research in this project is interested in exploring the features of the photorespiratory pathway that appear to buffer the smooth functioning of the “normal” carbon dioxide-fixing processes in the cell (i.e., the Calvin Cycle). This project seeks to identify the key metabolites in photorespiration that contribute to the protective roles, study details of their transport and their dynamics during transient environmental changes (e.g., sudden changes in light). This project also involves underappreciated metabolic exchanges between the chloroplast and other compartments, such as the newly uncovered glucose 6-phosphate “shunt” and potential novel sensors of inorganic carbon (i.e., CO2).
2. The chloroplast lipid membrane and its direct interactions with neighboring cellular machinery (Benning, Brandizzi, Hu)
The membranes of the chloroplast (particularly the inner and outer membranes) serve an important role in “gatekeeping” of what travels in and out of this compartment. This project is concerned with the flux and regeneration of chloroplast lipids and the precursor molecules that are used to build these membranes. Key areas of study also include the investigation of physical contacts between the membranes of the chloroplast and other neighboring cellular structures (mitochondria, peroxisome, endoplasmic reticulum). We seek to understand how these physical contacts may act as regulatory hubs to control transport and decide the fate of damaged chloroplasts.
3. Balancing the photosynthetic “energy budget” (Ducat, Sharkey, Walker)
Plants need to carefully balance the energy they obtain from absorbing light with their capacity to use that energy to perform cellular work (especially, to fix CO2 into sugars). When attempting to design more efficient photosynthetic processes, research usually focuses on the upstream light harvesting reactions that lead to “deposits” of fixed carbon in the cell. However, if the carbon that is fixed is not “withdrawn” in a timely manner, the metabolites of the Calvin Cycle can pile up, leading to a situation where the downstream reactions of metabolism constrain photosynthetic efficiencies. Questions in this project relate to how the chloroplast senses the correct amounts of carbon to withdraw and how the chloroplast rebalances its energy budget when the rate of withdrawal is not in sync with the rate of deposits.
4. Fine-tuning the chloroplast to environmental conditions (Howe, Hu, Strenkert, Walker)
For each of the topics areas above, the emphasis of understanding the balancing of photosynthetic processes throughout the cell is focused on the short-term (i.e., subsecond to hours). However, environmental challenges arise that present a longer-term obstacle (hours to seasons) to plants, such as a heat wave, nutrient deficiencies in the soil or a pathogen attack. In these circumstances, the priorities of the plant change and it must reallocate resources, such as increasing the energy spent to make defensive compounds that protect against a pathogen or grazer. The focus of these projects are on how the cell sends signals to the chloroplast that prepare it for non-ideal growing conditions, how these signals influence photosynthetic processes inside the chloroplast and lead to reallocation of carbon resources to respond to the threat.
This research is one of three core projects funded by the US Department of Energy, Office of Basic Energy Sciences