Project B: Optimizing photosynthetic productivity with subcellular and cellular modules
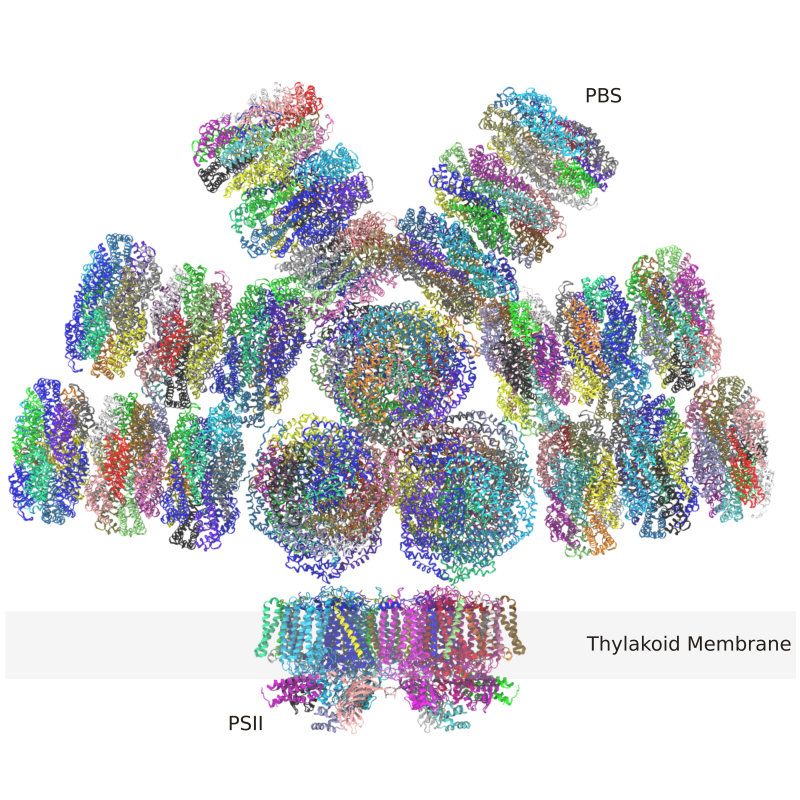
Image by Daipayan Sarkar
Photosynthesis as a process is modular, with different levels of modularity that open the door to engineering improvements that enhance photosynthesis. Photosynthesis within cyanobacteria specifically exemplifies this modularity. This is built from discrete modules for the light reactions (such as the phycobilisome and the photosystems) and CO2 fixation processes that are encapsulated by a specialized protein-based organelle, the carboxysome. Within this project, we are keenly interested in developing a mechanistic understanding of how individual modules operate so they can be better integrated into other photosynthetic organisms.
The key goals for our work exploring the modularity within photosynthesis are:
- Interrogate phycobilisome structure function relationships, both with other macromolecular modules such as the photosystems, and within the phycobilisome as an assembly with natural variation in its structure.
- Model and experimentally validate how the modular composition of the carboxysome shell influences its permeability and hence the catalytic activity of the encapsulated enzymes Rubisco and carbonic anhydrase.
- Develop engineering approaches to add alternative modules into new biological contexts, such as incorporating bacterial microcompartments into plant systems.
1. The phycobilisome and friends (Ducat, Kerfeld, Kramer, Vermaas)
The phycobilisome is the primary light harvesting complex in cyanobacteria and is itself a protein and pigment assembly with observed variation across photosynthetic bacteria. The basic architecture for the phycobilisome is a bi-, tri- or pentacylindirical core, with attached rods that harvest light energy. When captured, the energy is transmitted through the core to photosystems that are non-covalently attached to the phycobilisome. Rather than being static structures, recent cryo-electron microscopy data has demonstrated that there are multiple distinct conformations for the rods relative to the core of the phycobilisome. Together with pigment-bearing proteins, they may modulate quenching as environmental conditions transition from low to high light. With this as a backdrop, PRL researchers are carrying out research addressing the following:
-
- What are the functional differences between the alternative core architectures? What are the costs and benefits to a photosynthetic organism by choosing a specific phycobilisome core architecture?
- How easy is it for rods to switch from one conformational state to another? What are the timescales involved in conformational change, and could they match quenching rates?
- Beyond large scale structural transitions, protein-protein interactions between the phycobilisome and interaction partners such as photoprotective carotenoid proteins and photosystems are a major focus.
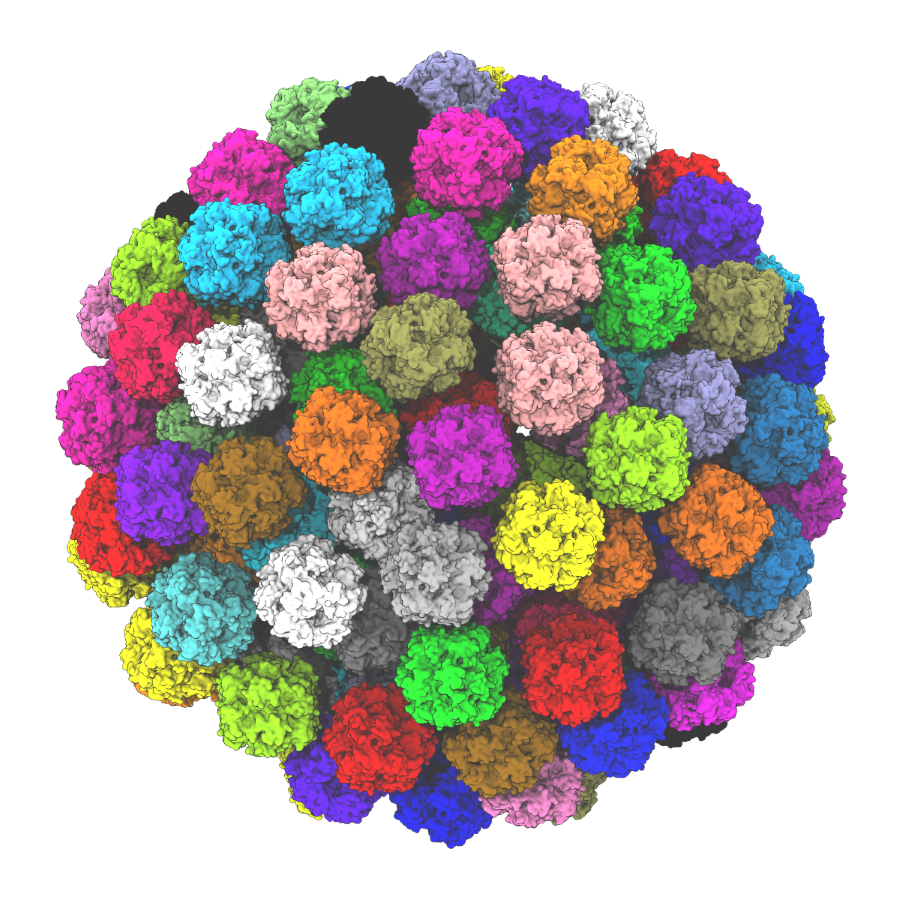
Image by Daipayan Sarkar
2. Carboxysome stories in transport and localization (Benning, Brandizzi, Froehlich, Ducat, Kerfeld, Vermaas, Walker)
The carboxysome is the prototypical photosynthetic module, where a proteinaceous shell encapsulates Rubisco and accessory enzymes such as carbonic anhydrase to improve the efficiency of photosynthesis in cyanobacteria. Our interest is in integrating carboxysomes with the rest of cellular metabolism, leveraging new tools for computational modeling and for probing structure and function at the mesoscale. At the heart of this investigation are the protein components that make up the shell, which are postulated to form a barrier that regulates the transport of photosynthetic metabolites. A longstanding hypothesis is that the size and charge of the pores within the hexameric and trimeric carboxysome shell proteins are tailored to keep oxygen away from Rubisco to reduce photorespiration, while allowing larger substrates such as ribulose bisphosphate access to Rubisco. The key questions that our research seeks to address are:
-
- Can we observe gas permeability predicted by molecular dynamics simulations via experimental methods?
- Ribulose bisphosphate is the largest compound that needs to traverse the carboxysome shell. How big is the barrier to transport for this molecule along the pores formed by hexameric and trimeric shell proteins?
- How does the carboxysome content and structure vary in a changing environment?
The carboxysome is the best characterized bacterial microcompartment (BMC). The modular character of BMCs lend itself well to potential synthetic biology applications. Ongoing work within the PRL is testing multiple mechanisms of introducing designed BMCs into plants. Two approaches being tested are transient expression of microcompartment components through genetic engineering approaches, or direct uptake of complete nanoscale BMC shells. By incorporating microcompartments into plants, our goal is to develop a platform to further tailor plant metabolism to house introduced biochemical reactions or deliver useful molecules like fertilizer into plant tissues.
This research is one of three core projects funded by the US Department of Energy, Office of Basic Energy Sciences.